Seeing That Which Cannot Be Seen
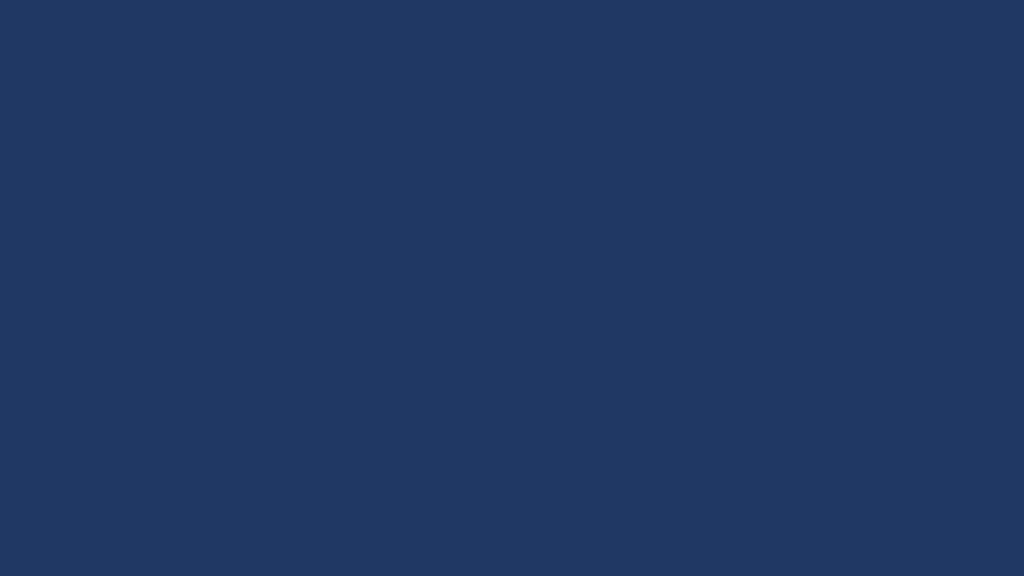
This article was a winning submission in an article writing contest held by the Astronomy Club at IIIT. Submission by Ujwal Narayanan
Introduction
I’ve always looked at the inky darkness of the night sky and wondered what lies beyond our planet. What wonders exist far away from our tiny little solar system. Quasars, clusters of stars orbiting each other, planets with two suns and red sands how marvellous would it be to see all of them. What’s even more amazing to me than everything you can see are the ones you cannot. I’m talking about black holes. Objects that are so dense that not even light can escape it.
Origins of Black Holes
Black holes are not new topics. John Michell first proposed the idea of a black hole in 1784. He hypothesized that electro-magnetic particles could not escape very massive objects and as such these bodies would be invisible. But with the wave nature of light coming out, the influence of gravity on waves was not well understood and as such interest dwindled.
Interested began once again with the arrival of Albert Einstein and his general theory of relativity. He conclusively showed that gravity can affect light, and ushered in the golden age of black hole research.
Initially the term “Dark Star” was used to describe this phenomenon. Later, the term “Black Hole” rose to prominence. The origins of this term are much closer home than many would imagine. It was inspired by a prison in Fort Williams, Calcutta called the Black Hole of Calcutta. People often joked that anybody who enters it would never leave. It was later used to describe black holes and the rest as they say is history.
Linguistic origins aside, let us now proceed to look at how they are actually formed. Black Holes are believed to be caused by the death of a massive star. We know that nothing can escape a black hole. Thus the escape velocity would have to be at least the speed of light. That is,
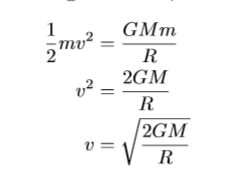
Substituting, v = c, we get
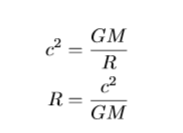
Thus, when this condition is satisfied a body becomes a black hole. Here
is the Universal Gravitational Constant, $R$ is the radius of the body and G
is its mass. M
For our sun ,for example, this radius often known as the “Schwarzschild radius” is around 3km. This means that if the sun ever shrinks to a size less than 6km in diameter it will become a black hole.
How massive would stars have to be for them to become black holes
Stars need to shrink beyond the Schwarzschild radius. They have to overcome the electrostatic repulsion between atoms to compress to a point of infinite density. Pauli’s exclusion principle also comes into play here, with it forbidding fremions like the electrons to occupy the same energy states. In this particular context, we term the force arising from this as \textbf{degeneracy pressure}. It was Chandrashekar who ventured to find the extent of this and as a result of his calculations, he found out that if a mass exceeds 1.4
where M
is the mass of the Sun, then gravity overcomes the degeneracy pressure. If it’s any less it won’t collapse into a black hole but will become a White Dwarf. M
What about Neutron Stars
Neutron stars are stars comprised mostly of neutrons. They have an outer shell comprised of neutron-rich while the inner parts are comprised of quantum fluid of neutrons. They seemed to violate Chandrashekar’s limit with stars having mass
> 1.4m
becoming neutron stars instead of black holes. This led to the formation of a new limit for neutron stars explained by the increased mass of the neutron when compared to that of the electron. Oppenheimer calculated this and fount out the limit to be roughly around M
. 3M
Observing the black holes
As nothing can escape the black hole, one of the ways we observe a black hole is by seeing how it affects the objects around it. One of the earliest known black holes was found in the Cygnus Constellation and by observing the way we receive light, the movement of stars around it, a black hole now called Cygnus X1 was identified.
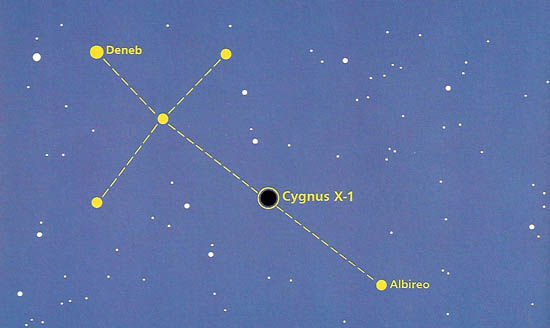
In 2015, Laser Interferometer Gravitational-Wave Observatory or as we know it LIGO discovered gravitational waves of two colliding black holes. This was a landmark discovery as it was the time we could directly observe a black hole.
This year is another special year in the history of black holes as for the first time we have pictures, not just radio waves or gravitational waves but a picture of a black hole.
Hole-y Picture
On Wednesday, 10th of April we captured the first photograph of a black hole. The team of researchers at Event Horizon Telescope worked tirelessly for a very long time to make this happen. But how exactly did they do it?
They began by imaging the biggest black hole silhouette in the sky. The black hole used is a supermassive black hole in the centre of the M87 galaxy. Even though it is one of the biggest black holes nearby, due to the distance, it appears extremely faint. To detect such faint images one would need a radio telescope with a dish the size of the earth.
It seems impossible to have a disk of this size. But using something called long baseline interferometry one can actually go about doing this. We create a virtual dish the size of the earth by coordinating numerous smaller dishes around the world. They all act in tandem to perform as a supermassive dish. For this particular image, radio telescopes from all parts of the world like Spain, Hawaii, Chile and even the South Pole was used, thus effectively giving it a radius approximately that of the size of the Earth.
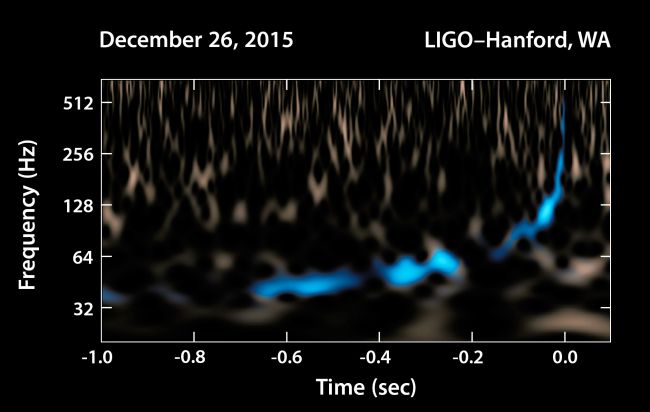
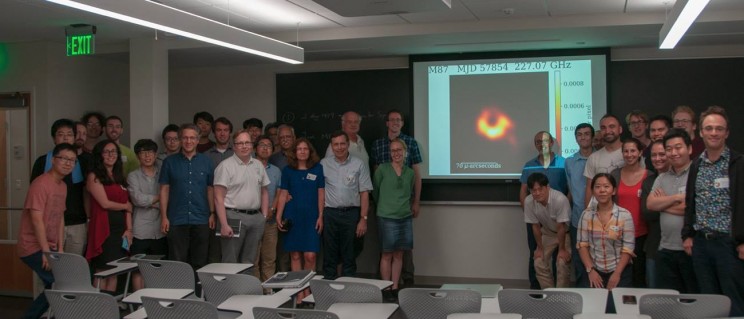
All these telescopes are pointed at the same point in the sky and data is collected. The data is then sent to a central server for image reconstruction. But this is not as easy as it sounds. Weather issues are an immense hassle when you are synchronizing across three continents. If in the occasions you do manage to sync up, you get terabytes of data every minute. How do you transfer all of it? The Internet alone is insufficient. So researchers took to manually shipping the hard drives across to MIT and the Max Planck Institute of Radio Astronomy in Germany. These places have special kinds of supercomputers called correlator which can be used to piece together these enormous quantities of data.
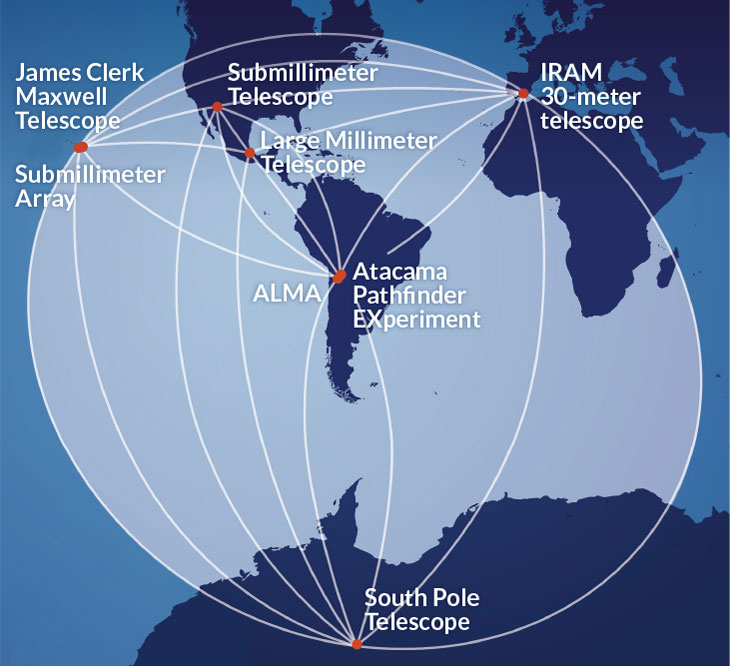
Efforts also had to be made to ensure that the reconstruction algorithm did not have any biases i.e it would not prefer one kind of structure over another. Through observations found by masking images and then proceeding to reconstruct them, the scientists managed to iron out most of the flaws in the algorithm.
All of these steps finally led to the image we’ve all come to know and love.

Other interesting things to consider
1.Describing a black hole
How does one differentiate between these bodies or even describe them? Science is all about quantifying things and measuring them. Saying that these are just black dense bodies won’t cut it.
Here we have theorem called the No hair theorem to aid us. It states that all black holes are the solutions of the Einstein-Maxwell equations and thus have only three properties, namely:
1. Mass
2. Radius
3. Angular Momentum
Any other property is behind the event horizon and thus we cannot observe it. Hair is used as a metaphor for information. It represents the features used to describe the features of the body, and no hair aptly describes how so little of the features are visible to us.
2. Can nothing escape a black hole
Quantum theory states that information can never be destroyed, yet general relativity predicts this is what’s happening when particles enter black holes. This paradox is termed as the \textbf{information paradox} and was made famous by Dr. Hawking. He postulated that information was not stored at the centre of the black hole but at its event horizon. Hawking also proposed that a temporary change in energy that occurs at a black hole could allow particles to escape as Hawking Radiation. These particles could then meet the bubbles of information stored at the event horizon and carry them out of the black hole, thus resolving the information paradox. While the actual particle itself might be destroyed, the information that the particle had, or it’s blueprint still exists and this what the Hawking Radiation carries. While information is escaping the black hole it is shuffled and is in a chaotic form and for all intents and purposes practically useless to us.

Black magic
Black Holes can also give the illusion o time stopping. Relativity establishes that time is not invariant. Due to the massive gravitational potential of a black hole, for an observer standing outside an event horizon watching somebody fall in, it would appear that time is slowing down for the body that is falling in. Time would get slower and slower until eventually, it appears that time stops.
Say if light with a frequency v
falls in. Then it has a change in its gravitational potential. Say it falls all the way to the centre. From de Broglie’s equations, we know that mc^2 = hv
. Therefore calculating the change in the frequency of light due to it falling into the centre, we have
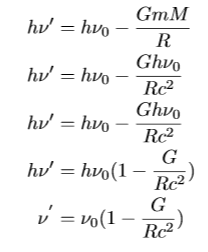
But at the event horizon,
becomes zero as no light can escape it. Thus the time v'
T
→
. Thus for an outsider outside it appears that time has stopped. This is not a completely accurate description as it uses the Newtonian model instead of the Relativistic one for Gravity, but the argument is similar in either case0
Conclusion
Black holes are one of the most fascinating objects in the universe. The creation of this and perhaps even the death of this universe are all entwined these dense objects. Yet we know so little about them. Research is underway and hopefully within the next decade or so we understand these dark bodies better and thus better appreciate the world around us and celebrate the wonder that is this universe.